About the laboratory
Material Research Laboratory was established by professor Matjaz Valant in 2009 and has evolved in a sizeable research unit with state-of-the-art equipment and diverse expertise of the team members ranging from synthetic and crystal chemistry, functional materials, surface science, theoretical and computational chemistry etc. We have not only maintained the initial research focus on environmental and electronic materials but also developed it towards new exciting and advanced material systems and processes that include topological insulators, nanostructured photo-catalysts, materials in extreme environments and energy storage technologies. The joint efforts of the team members resulted in some exciting discoveries and developments. Our highly esteemed research work enables us to work with the most important academic and industrial laboratories all over the world.
Topological insulators
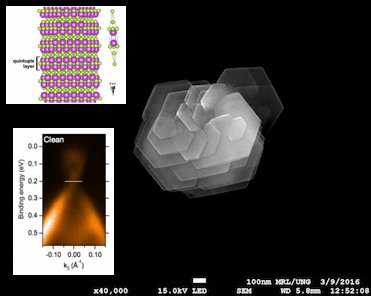
Topological insulators (TI) are special kind of materials that behave as insulators in its interior and have conductive surface because of present topological conductive states. We are focusing on several chemical-related studies including a chemical and electronic interaction between topological insulators and metal contacts, stability of topological surface state against chemical modifications, defect chemistry of TI etc.
Topological insulators nanoaprticles can be prepared by using various methods. However, we discovered, that TI, prepared in the present of a organic molecule have different optical and photo-thermal properties, compared to the particles prepared in water. The properties of the TI nanoparticles can be also modified by their composition – adding dopants. On this basis, we can fabricate various doped TI and follow their optical response, which originates in the topological surface states, with UV-VIS spectroscopy. We are developing the first direct analytical method, that can tell us whether the nanoparticles are topological insulators or not, using a common technique that is available in almost every laboratory in the world. Up till now, detection of TSS is possible only for bulk materials and by using ARPES method on synchrotron.
Grid energy storage
The shift to renewable sources of energy is beginning to expose a fundamental weakness in the world’s electricity grids. Solar, wind, wave and other forms of renewable energy are all, to some extent, subject to the vagaries of factors like the weather. This results in a fact we cannot match the amounts of energy available with demand, because we do not have an effective way to store and then release this energy when we need it. Globally speaking, the problem is not a shortage of energy; it is primarily one of efficient energy storage. Existing energy-storage systems all suffer fundamental weaknesses: environmental and economical drawbacks, like batteries and energy-to-flue technologies; they are geographically limited, like pumped storage hydropower or they suffer from low efficiency, like compressed-air energy storage. We need a storage method that overcomes all the above limitations.
Based on our patented concept we are developing a completely new grid energy storage technology that can be classified as a power-to-solid approach. It has a potential to store energy with a much higher density than the power-to-gas or power-to-liquid approaches. The reason for this is that matter is packed much more densely in a crystalline solid than in a gas or liquid.
Heterogenous CO2 hydrogenation by modification of active site environment
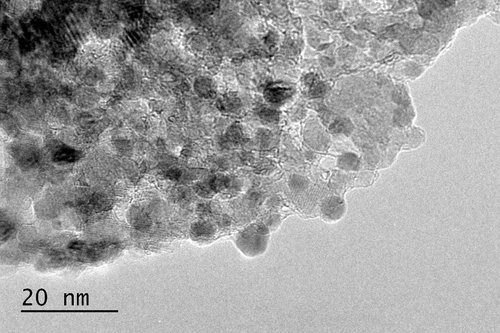
The path towards CO2 neutrality needs to go over the reduction of CO2 emissions. Consequently, techniques as on-site CO2 capture and CO2 conversion to fuels and chemicals are of extreme interest. Due to the high energy that is required for the activation of CO2 molecules a product-selective and robust catalyst has to be developed and applied. Of particular interest is the formation of C2+ products (ethanol, ethane, ethylene, ethylene glycol…) that are carrying higher energy density and higher economic value compared to the C1 products (methanol, formic acid, methane…). Until now, only the methanol production from CO2 has reached technological maturity with sufficient selectivity. Whereas, the C2+ products are usually formed with low reaction yields mostly as side products of the C1 products. A significant breakthrough in the selectivity of products from the CO2 reduction can be achieved only by understanding the reaction mechanisms, surface structures, and active sites. We are addressing the selectivity issue by modification of catalytically active sites. For thermally driven CO2 hydrogenation, we are studying the potential of grain boundaries and crystallinity in hydrotalcite-derived metal oxides to disperse active metal sites. For electrochemically driven CO2 hydrogenation we are developing electrodes modified by a porous organic framework. This allows confining CO2 gas and reaction intermediates, forcing the C-C bond formation.
Hydrogen-generating photocatalytic materials and processes
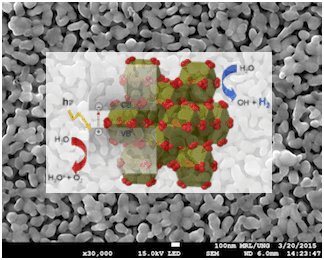
Hydrogen can be generated by splitting water molecules on a photocatalysts or an electrode, or as a product of ceratin chemical reactions . Within our research we are developing new photocatalytic materials, study their structural, electronic and photocatalytic properties and the influence of morphology, composition and particle size on the photocatalytic activity. In addition, we are studying new hydrogen-producing photocatalytic reactions and processes as well as photoelectrochemical processes for simultaneous water purification and hydrogen generation.
Computational solid-state chemistry
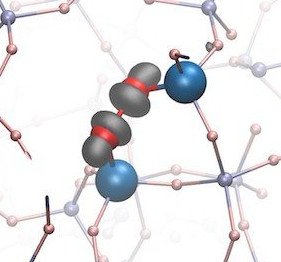
Simulations based on density functional theory (DFT) have become an extremely successful tool for describing the ground state properties of a wide range of bulk and more complex form of matter such as nanostructures and interfaces and also for calculation of activation energies of chemical reactions. For simulations of optical properties we use the most advanced and accurate approaches based on combination of GW method and Beth-Salpeter equation. With methods mentioned above we solve problems like defects on atomic scale in amorphous silicon and oxidation of metal surfaces.
Use of the reductive potential of algae
Objective of the projects are development of new green technologies for metallization of polymeric surfaces and for remediation of metal polluted wastewaters by reductive potential of algae.
Metallization by algae does not require expensive industrial facilities, which are adapted to conventional vacuum loading. Additionally it completely removes the use of toxic and aggressive chemicals that have a big influence on the environment. Another advantage is that for metallization there is no need for high temperatures as is for vacuum loading since the processes are carried out at room temperature. Because of this also the use of energy is reduced.
Systems with hydrogen producing algae will be used for research of biological remediation of waters polluted with metal ions. Obtained knowledge will contribute to better understanding and more efficient removal and recycling of metal ions from wastewaters.
Project is co-financed by Republic of Slovenia and European Union (European Regional Development Fund).
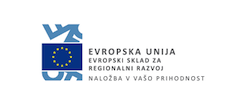
